Research
Research in our laboratory is focused on mammalian germ line stem cell biology. Germ line stem cells (spermatogonial stem cells) form the basis of male fertility and are the only cells in an adult body that divide and can contribute genes to subsequent generations, making them immediate targets for genetic manipulation. There also appears to be considerable plasticity between germ line stem cells and pluripotent stem cells, indicating the potential to use germ line stem cells for regenerative medicine.
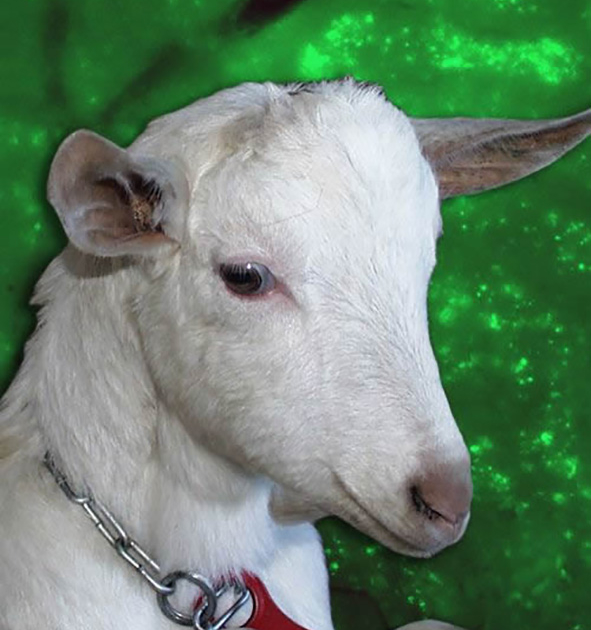
We were first to apply germ line stem cell transplantation in non-rodent models to transmit a genetic change introduced into germ line stem cells to the next generation (FASEB J. 2008; Figure 1) and have more recently extended this work to a porcine model (Biol. Reprod. 2013).
Figure 1(Left). The foreground image shows one recipient goat that received the transplantation of GFP-positive germ cells into the testis. The background image shows the fluorescent testis after germ cell transplantation.
Study of human and animal disease requires appropriate models. While there are many rodent models, larger animal models such as pigs more closely represent human physiology, size and longevity. Our work enables generation of genetically modified non-rodent animal models that is potentially more efficient than the current approaches (Figure 2).
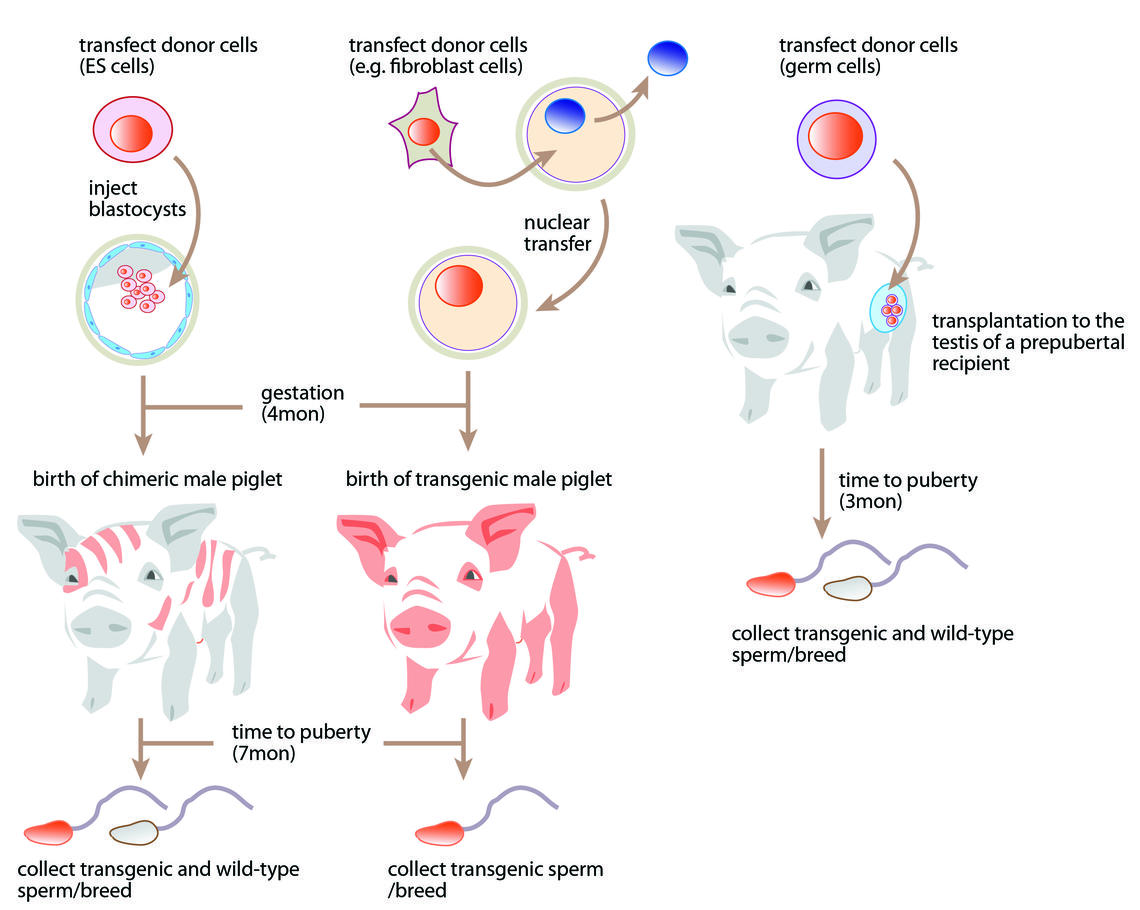
Figure 2. Schematic comparison of different approaches to transgenesis in pigs. Left: transduction of embryonic stem cells and chimera production by blastocyst injection (modeled on the rodent system, not yet demonstrated in pigs); middle: transduction of somatic cells followed by somatic cell nuclear transfer (current state of the art); right: transduction of germ cells followed by germ cell transplantation. Transgenesis through the male germ line is potentially more efficient than the alternate approaches shown (Anim. Reprod. 2015).
As a complementary approach to germ cell transplantation, we developed xenografting of testis tissue as an accessible in vivo system to study germ line stem cells and spermatogenesis (Nature 2002; Figure 3). This technique makes it possible to produce sperm from immature males and allows controlled experimentation in donor species such as primates where experiments in whole animals would be logistically and ethically difficult (Biol Reprod. 2004; Endocrinology. 2008). With this model we demonstrated a deterimental effect of chronic exposure to phthalate esters on the developing primate testis (Mol. Cell. Endocrinol. 2005).
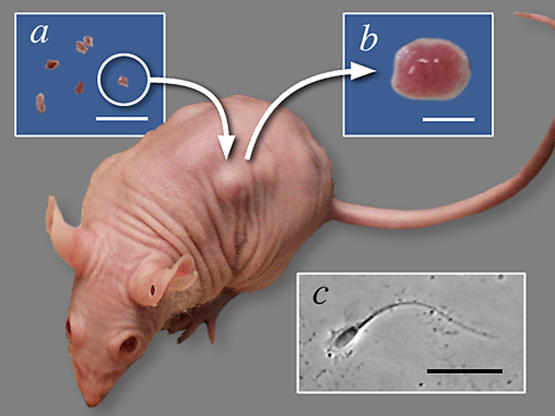
Figure 3 (left). Grafting of testis tissue from newborn piglets under the skin of nude mice. a, b, The size of the pig testis grafts at the time of transplantation was about 0.5–1 mm in diameter (a) and expanded to 4–8 mm at 10 weeks after grafting (b). Scale bars, 5 mm. Most grafts from later time points contained sperm. c, A sperm extracted from a week 27 graft. Scale bar, 20um (Nature 2002).
Extending the versatility of this approach to allow manipulation of different cell types prior to grafting, we subsequently established de novo morphogenesis of testis tissue from single cell suspensions grafted ectopically into mouse hosts (Biol. Reprod. 2007; Organogenesis. 2007; Figure 4).
Figure 4 (below). Schematic representation of de novo morphogenesis of testis tissue from isolated cells grafted into a mouse host. A single cell suspension obtained from immature donor testis is grafted ectopically to a mouse host. Testis tissue formed de novo from the grafted cells supports spermatogenesis. De novo morphogenesis of testis tissue is based on the ability of different testicular cell types to rearrange after grafting into functional cell associations (Cell & Tissue Res. 2012).
In addition to providing platforms for the study and manipulation of male germ line stem cells, these transplantation approaches also have applications for preservation of fertility (Figure 5 - see below).
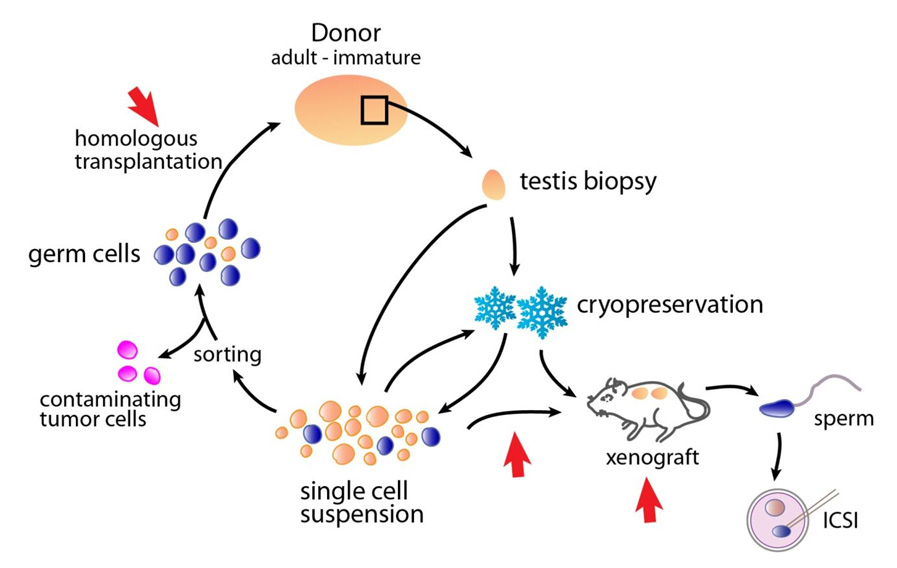
Figure 5. Schematic representation of cell or tissue transplantation to preserve male fertility. From left to right red arrows indicate germ cell transplantation to a host testis, de novo morphogenesis of testis tissue after xenografting of a single cell suspension, and xenografting of testis tissue to generate fertilization competent sperm.
Building on our previous discoveries, we currently focus in four areas of research:
To enable efficient manipulation of germ line stem cells prior to transplantation, large scale enrichment of non-rodent GSCs is required. We refined strategies for cell isolation based on differential adhesion properties both in static culture and in stirred suspension culture (Andrology,2015). We are now exploring suspension culture in stirred bioreactors, shown to support expansion of pluripotent stem cells (Stem Cells & Dev., 2019), for its utility to support GSC proliferation.
We demonstrated previously that viral- or non-viral- mediated transfection prior to transplantation resulted in germ cell transmission of a marker gene (gain-of-function, random integration) by recipient males. We are now extending the approach to induce loss-of-function mutations through targeted deletions by introducing TALEN (Mol. Reprod. Dev., 2018) and CRISPR/Cas9 constructs into germ cells followed by transplantation to recipient pigs to generate large animal models of human disease.
We observed that spermatogonia in situ show low levels of autophagy, yet germ cell autophagy increases with removal from the testicular microenvironment and exposure to stressors such as increasing levels of reactive oxygen species or reproductive toxicants such as phthalate esters (Env. Epig., 2017). We are currently investigating the function of autophagy in germ cell homeostasis. Findings may aid in developing improved conditions to propagate germ cells in vitro.
De novo formation of functional testis tissue from isolated cells transplanted into a mouse host provides an accessible in vivo system to study testis function. We recently developed organotypic testicular organoids as an in vitro platform to study cell-cell interactions in the mammalian testis (Biol. Reprod., 2019). We demonstrated the presence of primary cilia on porcine Sertoli cells during testis development and are exploring their function (Cell Tiss. Res. 2014, 2017, 2020). We are also studying the ability of the de novo formed testicular microenvironment to support differentiation of germ cells derived from induced pluripotent stem (iPS) cells.